A propulsion system, in general, is the generation of force to ‘propel’, typically, a rigid body.
Rocket propulsion systems are the mechanisms & technologies used to propel rockets and are the driving force of space exploration.
These complex systems are responsible for generating the immense amounts of thrust needed to propel rockets and spacecraft into orbit and beyond.
The history of rocket propulsion dates back to ancient times when people used simple rockets powered by black powder to create fireworks and signals.
The modern era of rocket propulsion began in the early 20th century when engineers and scientists started to develop more powerful and sophisticated rocket engines.
Today, rocket propulsion systems are a crucial part of space exploration and satellite technology.
Propulsion systems are used to launch spacecraft into orbit, to send probes and landers to other planets, and to power satellite and space station systems.
Principles of Rocket Propulsion
The principles of propulsion systems are based on the concept of generating thrust, or force, to propel a rocket or spacecraft through space.
This can be achieved by burning fuel and an oxidizer in a combustion chamber, creating hot gases that are forced out of the rocket through a nozzle.
The reaction between the fuel and oxidizer generates a massive amount of force, or thrust, which propels the rocket forward.
The design and operation of rocket propulsion systems is a complex and highly specialized field.
Engineers must carefully balance the fuel and oxidizer proportions, the size and shape of the combustion chamber and nozzle, and the amount of thrust generated in order to create a propulsion system that is efficient, powerful, and reliable.
Rocket principles are based on Newton’s laws of motion and describe the forces acting on a rocket and the resulting motion of the rocket.
Briefly, following are the Newton’s three laws of motion:
- Objects at rest will stay at rest and objects in motion will stay in motion in a straight line unless acted upon by an unbalanced force.
- A force applied on an object will accelerate it and the rate of change of momentum of the object is equal to the applied force.
- For every action, there is always an equal and opposite reaction.
The principles of propulsion systems can be understood in terms of Newton’s laws of motion.
Newton’s First Law in Rocket Propulsion
Newton’s first law of motion states that an object will remain at rest or in motion at a constant velocity unless acted upon by an external force.
It helps us understand the basic principles of how a rocket is able to lift off the ground and achieve lift.
A rocket is initially stationary and therefore subject to the force of gravity pulling it down toward the Earth.
The force of the rocket’s exhaust gases pushing against the rocket’s nozzle creates an equal and opposite reaction force, which creates an unbalanced forced on the rocket that propels it upwards and overcomes gravity.
When a rocket is in motion, it will continue in a straight line unless acted upon by an external force.
This means that if the rocket’s engines are not firing or if the rocket is not being affected by external forces, it will maintain a constant velocity and follow a predictable path.
When a rocket is moving through the air, it will experience a force of air resistance pushing against it, which can alter its motion and trajectory.
- The first law of motion can be used to predict the trajectory and stability of a rocket in flight.
- It can be used to understand the effects of external forces, such as air resistance, on a rocket’s trajectory and performance.
- Lastly, it can help us calculate the effects of these forces and to determine necessary adjustments to the rocket’s engines or flight path.
Newton’s Second Law in Rocket Propulsion
Newton’s second law states that the rate of change of momentum of an object is directly proportional to the force acting on it and in the same direction.
In the case of a propulsion system, the force acting on the rocket is the thrust generated by the propulsion system.
The greater the force, the greater the acceleration of the rocket.
The amount of thrust generated by a propulsion system is directly proportional to the mass flow rate of the propellants and the exhaust velocity of the hot gases.
This means that the more propellant that is burned and the faster the hot gases are expelled from the rocket, the more thrust will be generated.
The second law of motion helps us understand the mechanisms of how a rocket is able to generate thrust and accelerate through the air.
The force of the rocket’s exhaust gases pushing against the rocket’s nozzle creates a reaction force that propels the rocket forward, according to the second law of motion.
This information is crucial for determining the size and design of the rocket’s engines and fuel system.
The law can also be used to predict the effects of external forces, such as air resistance, on a rocket’s trajectory and performance.
This knowledge is important for ensuring the safety and accuracy of rocket launches.
- The second law of motion helps us understand the mechanisms of how a rocket generates thrust and accelerates through the air.
- It can be used to calculate the required force (or thrust) needed to accelerate a rocket to a specific velocity or reach a certain altitude.
- Information about the required thrust is crucial for determining the size and design of the rocket’s engines and fuel system.
- Also, we can use it to predict the effects of external forces, such as air resistance, on a rocket’s trajectory and performance, which is important for ensuring the safety and accuracy of rocket launches.
Newton’s Third Law in Rocket Propulsion
Newton’s third law states that for every action, there is an equal and opposite reaction.
In the case of a propulsion system, the action is the expulsion of hot gases out of the rocket’s nozzle, and the reaction is the thrust that propels the rocket upward.
The magnitude of the thrust is determined by the mass and velocity of the hot gases expelled from the rocket.
The direction of the thrust generated by a propulsion system can be controlled using thrust vectoring, which involves adjusting the orientation of the nozzle
This allows the rocket to control its orientation and direction of travel in space.
By adjusting the angle of the rocket’s nozzle or using movable fins or nozzles to direct the flow of hot gases, the direction of the thrust can be changed, allowing the rocket to steer and change its direction of motion.
- The third law of motion helps us understand the basic principles of how a rocket generates thrust and accelerates through the air.
- It can be used to predict the behavior and stability of a rocket in flight.
- It can be used to calculate the effects of these forces and determine necessary adjustments to the rocket’s engines or flight path.
- The law also helps us understand the mechanics of thrust vectoring, which is the process of controlling the direction of the thrust generated by a propulsion system.
Propulsion System’s Efficiency & Performance
A rocket’s main components are its engines, propellant tanks, payload, control system, and propellants while propellants are typically the heaviest part of the rocket.
The type of propellant used in a propulsion system has a major impact on its performance and efficiency.
Different propellants have different energy densities, burn rates, and exhaust velocities, which can affect the amount of thrust generated and the specific impulse of the propulsion system.
The efficiency of a propulsion system is determined by the specific impulse, which is the amount of thrust generated per unit of propellant burned.
So a good propellant choice will have a higher specific impulse which will make a more efficient propulsion system.
All three of Newton’s laws of motion are relevant to the design of efficient propulsion systems.
Types of Rocket Propulsion Systems
Rocket propulsion systems can be classified based on the type of fuel or power source they use:
Chemical Rocket Engines
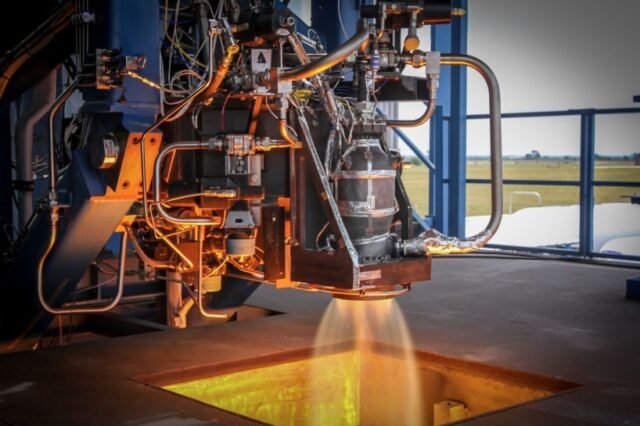
A chemical rocket engine uses a chemical reaction between a fuel and an oxidizer to produce hot gases that are expelled through a nozzle to generate thrust.
The most common type of chemical rocket engine is a liquid rocket engine, which uses liquid fuels and oxidizers, such as kerosene and liquid oxygen.
When the fuel and oxidizer are mixed and ignited, they undergo a chemical reaction that produces hot gases, which are then expelled through the nozzle to generate thrust.
Another type of chemical rocket engine is a solid rocket engine, which uses solid fuels and oxidizers, such as rubber and ammonium perchlorate.
In a solid rocket engine, the fuel and oxidizer are mixed together and packed into a solid rocket motor, which is then ignited to produce hot gases which are then expelled through the nozzle to generate thrust.
Chemical rocket engines are the most commonly used type of rocket engine, due to their high thrust and relatively simple design.
However, they have some limitations, such as a limited amount of fuel and oxidizer that can be stored onboard, and a lower specific impulse compared to other types of rocket engines.
Examples of chemical rocket engines include liquid rocket engines, such as the Merlin engine used on the SpaceX Falcon 9 rocket, and solid rocket engines, such as the Space Shuttle Solid Rocket Boosters.
Nuclear Thermal Rocket Engines
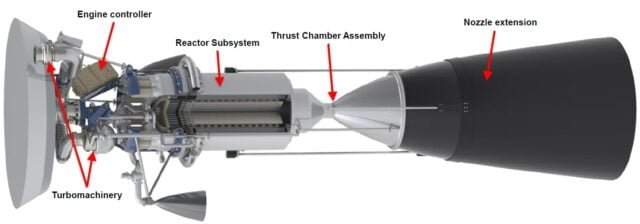
A nuclear rocket engine is a type of rocket engine that uses nuclear reaction as its source of energy.
These engines use nuclear reactions to heat a working fluid, which is then expelled through a nozzle to generate thrust.
This type of engine is much more powerful than traditional chemical rockets and is capable of reaching much higher speeds.
Unlike chemical rockets, which carry their own oxygen supply to burn fuel, a nuclear rocket uses the engine itself to create the heat and pressure needed to generate thrust.
This means that a nuclear rocket engine can operate in the vacuum of space, where there is no air to provide oxygen for combustion and it’s not required to carry an oxidizer.
However, nuclear rocket engines are much more complex and expensive to build and operate than chemical rockets and have not yet been widely used in space exploration.
The NERVA engine developed by the U.S. government in the 1960s and the PROPULSE engine proposed by NASA for future Mars missions are some examples of Nuclear Thermal Engines.
Electric Propulsion Systems
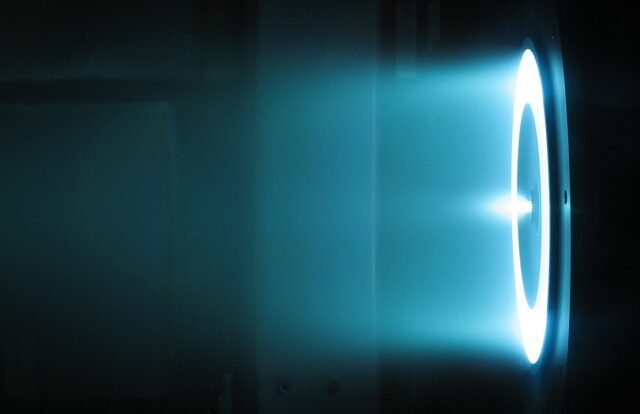
Electric propulsion (EP) systems are a type of spacecraft propulsion that uses electric power to accelerate a propellant.
The electricity is typically provided by a solar panel or a nuclear reactor and can be used to heat the propellant, ionize it, or directly accelerate it using an electric field.
This allows the spacecraft to achieve high speeds with relatively low amounts of propellant, making EP systems well-suited for long-duration missions or missions that require a large change in velocity.
There are several different types of EP systems, including ion thrusters, Hall thrusters, and plasma thrusters.
These systems are often used for spacecraft that do not need to carry a large amount of propellants, such as satellites or interplanetary probes.
Some examples of electric propulsion systems include ion engines, such as the Hall-effect thrusters used on the Dawn spacecraft, and solar-electric propulsion systems, such as the one used on the Deep Space 1 mission.
Hybrid Rocket Engines
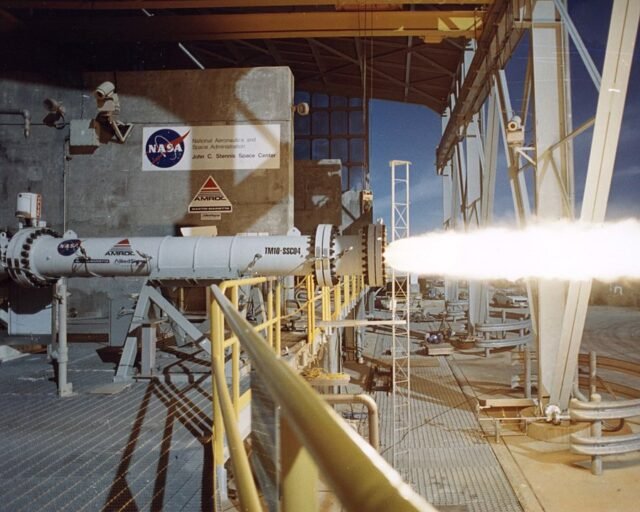
These engines combine features of both chemical and solid rocket engines, using a solid fuel and a liquid or gaseous oxidizer.
In a hybrid rocket, the fuel is typically a solid material, such as rubber or a synthetic polymer, while the oxidizer is a liquid or gas, such as nitrous oxide or hydrogen peroxide.
When the fuel and oxidizer are combined, they undergo a controlled chemical reaction that produces hot gases, which are then exhausted through a nozzle to generate thrust.
One advantage of hybrid rocket engines is that they can be easily shut down and restarted, which is not possible with solid or liquid rocket engines.
This makes them well-suited for use in launch vehicles, where the ability to abort a mission is important.
Hybrid rocket engines are also relatively simple and inexpensive to manufacture and operate, which makes them attractive for use in small satellites and other spacecraft.
However, they are not as powerful as other types of rocket engines and are typically not used for high-speed or long-duration missions.
The SpaceShipOne rocket that uses a solid rubber fuel and nitrous oxide oxidizer, and the Rocket Lab Electron rocket that uses a solid plastic fuel and liquid oxygen oxidizer are some examples of Hybrid Rocket Engines.
Recommendations
Pranav Mahapatra and Devesh Sharma, ‘How do Rockets Work‘, Evincism