Twinkle twinkle little star,
How I wonder what you are.
Honestly, this is one of the most ironic poems ever written, which is still taught at kindergartens.
In a limited sense, the poem is right, but the truth is that the stars are humongous celestial bodies.
One cannot simply imagine how big, how bright, or how interesting the stars can be.
So if you ever wonder about stars like what they do, and why they are the way they are, you’re just at the right place.
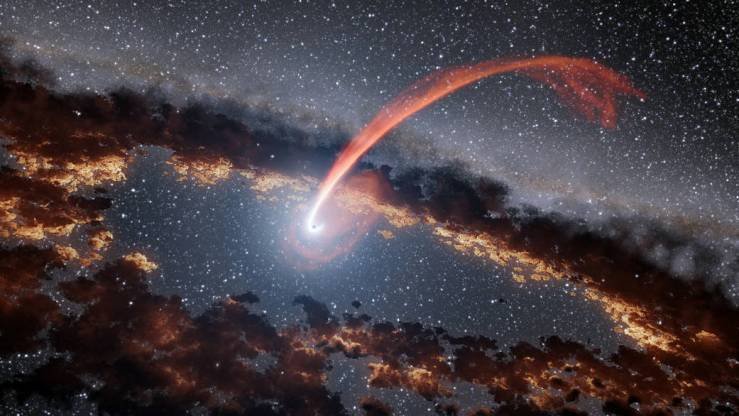
Stars have their place among the most magnificent objects in the Universe.
They literally give life to the universe as they are the major and most widespread sources of energy across the cosmos.
If there were no stars, then the entire universe would’ve just been in darkness and there would be no life at all, but fortunately, stars do exist; exposing the wonders lurking within our universe.
So it’s worth sparing some time to talk and learn about stars.
History of Our Understanding of Stars
Stars have been decorating the night sky since the beginning of the universe, and it turns out that our ancestors were quite curious about them.
In ancient times, people actively studied stars and their positions.
They identified certain patterns among the twinkling dots and named them, these patterns are what we now call constellations.
Astronomy (study of stars) is, surprisingly, older than Physics because ancient people had an odd zeal to study the stars more sturdily than the laws of nature.
The positions of stars helped them navigate themselves throughout a long journey.
Knowledge of the positions of the stars and constellations also helped them to keep a record of time.
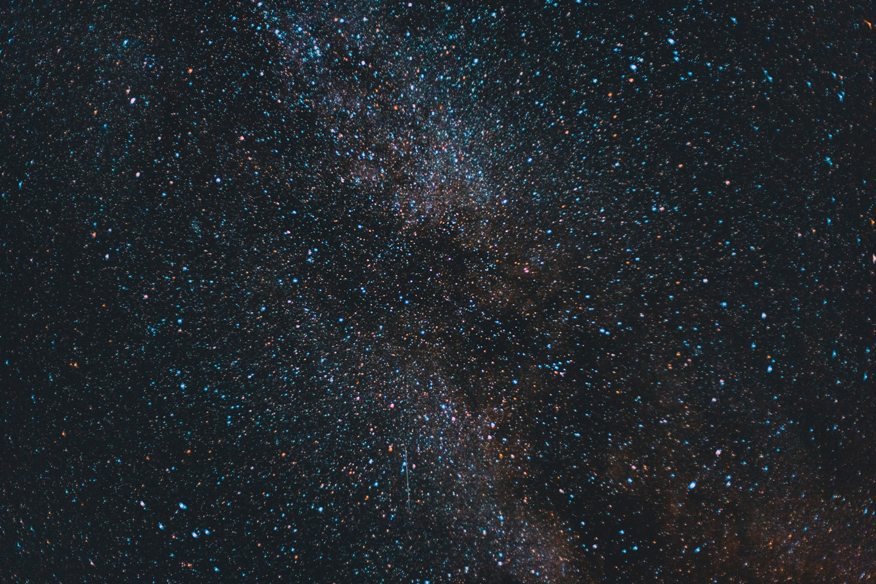
Ancient Babylonian, Assyrian, Egyptian, Indian, and Chinese were known for studying space and stars for roughly 3000 years, while some also maintained their records.
The Chinese also recorded comets, bright meteors, and dark spots on the Sun.
Later, Chinese astronomers kept careful records of “guest stars” — those that are normally too faint to see, but sometimes (suddenly) flare up to become visible for a few weeks or months.
We still use some of these existing records for studying stars that exploded a long time ago.
Now, astronomy is not only about constellations and their positions.
Modern astronomy focuses on the classification of objects in the night sky and studying various stellar events such as the formation of stars, supernovae (death of stars), white dwarfs, brown dwarfs, exoplanets, black holes, neutron stars, comets, and asteroids.
We’ll talk about all of those mentioned above but let us first understand what is a star, typically.
What is a Star?
Stars are massive, self-luminous celestial bodies made up of gasses that shine by emitting radiation produced inside their cores.
Stars release energy through fusion reactions that start with fusing hydrogen atoms (the main constituent of stars) to produce helium (the second-most abundant element in stars).
It is the same reaction that takes place inside a Hydrogen Bomb.
We can refer to these stars as huge hot balls of hydrogen, helium, and other elements as well.
How is a Star Formed?
Stars are formed due to the accretion of molecular gasses and dust in nebulae (or molecular gas clouds).
A nebula (plural nebulae) is an unimaginably huge interstellar cloud of dust and gasses like hydrogen, helium, and other ionized gasses.
An average nebula is around 100 light-years in diameter and mostly consists of hydrogen gas, which is the most essential component for the formation of stars.
Due to the mutual attraction and certain disturbances, the dust and gasses start gathering at one point.
This increases the mass (and thus, gravity) at that point, which further accelerates this accumulation.

Now, the dust and gasses start revolving around the newly integrated central mass, form a disc, and continue crowding in.
Once enough matter is accumulated from the surrounding, it starts producing heat, forming a hot core at the center.
This is the initial stage of the star where it is called a Protostar or Prostar – a very young star.
When the collection becomes large and dense, it continues to grow until its density, pressure, and temperature becomes favorable to ignite a fusion reaction in it.
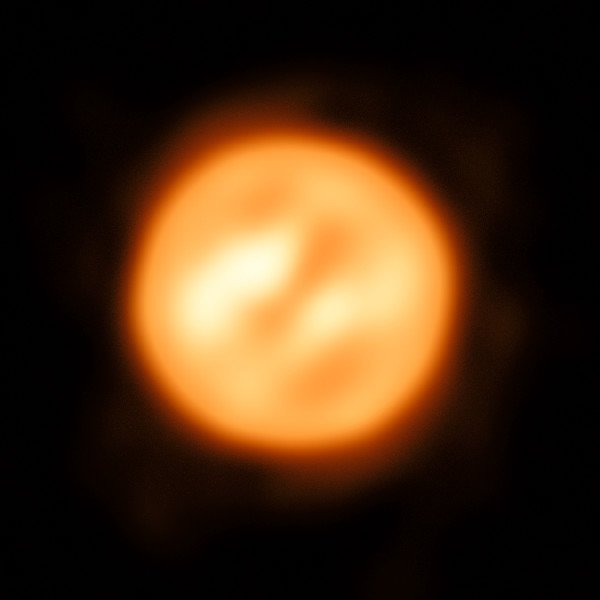
During a fusion reaction, hydrogen atoms collide violently to combine and form helium atoms in the process.
Quantum Tunneling plays an important role in combining hydrogen atoms.
Once the fusion reaction starts, a shock wave of solar wind is produced which sweeps away the excess gasses and dust.
These cosmic remnants that could not become a part of the star don’t go waste (not all of it, at least). They accumulate to form planets, asteroids, and comets.
This was how our planet (a rotating accumulation of dust around its parent star – the Sun) and solar system came into being.
A star is sustained over billions of years by the perfect balance of pressure inside it.
The core of the star is dense and wants to draw the entire star toward itself.
As the elements inside a star fuse into heavier ones, they release an outflow of energy in the form of heat and light.
This outflowing pressure, due to the energy produced, counterbalances the inward gravitational pull of the core, thus preventing the star from either collapsing or inflating.
Now the question that arises is what happens when there are no more atoms to fuse.
That is when the collapse becomes inevitable because there is no outward pressure to counter it.
The star implodes and dies, leaving behind… well, what it leaves behind depends on the star.
Different stars work differently and meet different fates. We’ll learn more about the same later in the article.
Do Stars Actually Burn?
If you want to shine like a sun, first burn like a sun.
A.P.J Abdul Kalam, Ex-Indian President & Former Scientist
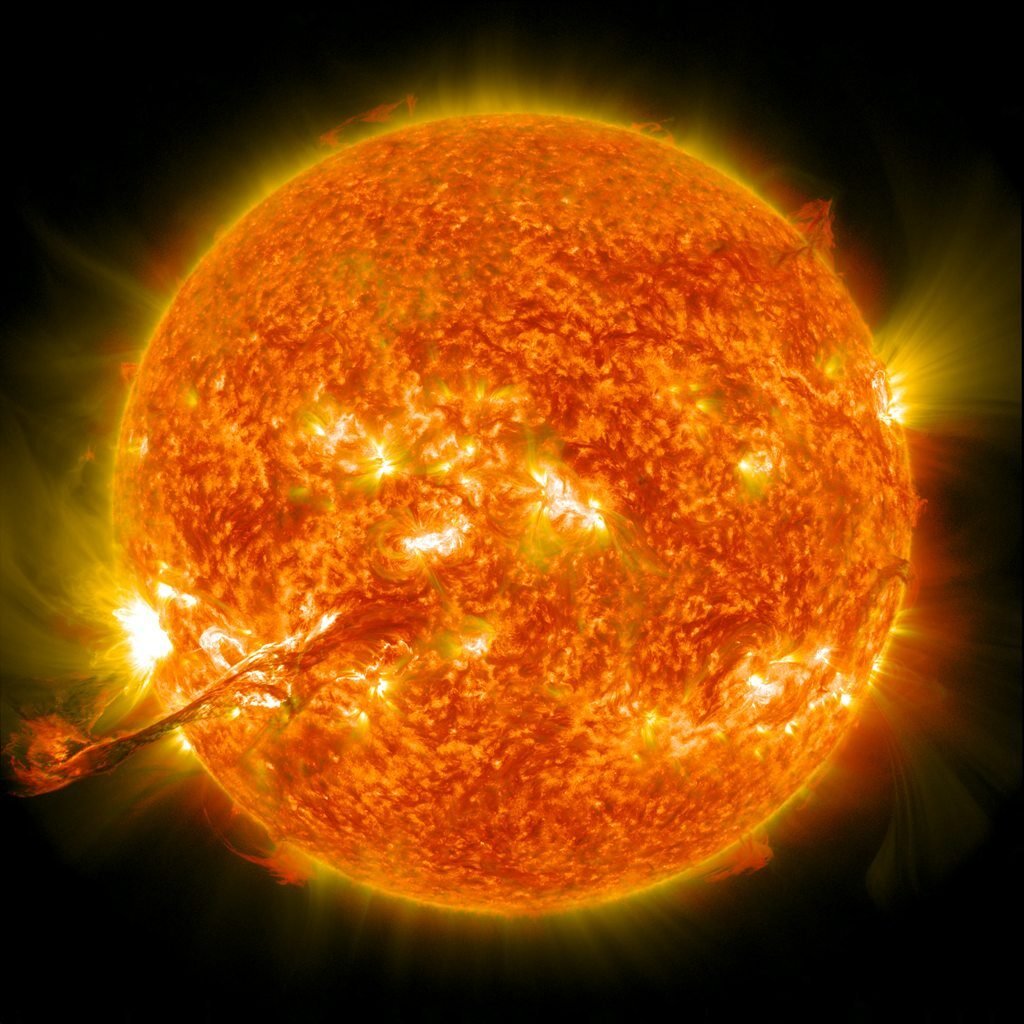
One of the most widespread misconceptions about stars is that they burn. Due to this, people often imagine stars, especially the sun as burning fireballs.
First of all, burning or combustion (the scientific word for burning) is a chemical reaction that happens in the presence of oxygen, where matter (a fuel) reacts with oxygen to form smoke.
This is very different from what happens inside stars.
Atoms inside a star undergo fusion reactions – a process in which two or more light nuclei fuse to form a heavier nucleus.
In stars, it is, initially, the hydrogen atoms that combine and form deuterium (an isotope of hydrogen) and helium, this process releases a huge amount of energy and light.
So technically, stars do shine, but they do not burn.
Where Do Stars Get Their Energy From?
You might say that the main source of energy in stars is nuclear fusion, right? Right; but how does this process of fusion release such huge amounts of energy? Comes Albert Einstein to the rescue!
You might be familiar with Einstein’s famous mass-energy equivalence principle, the $\:E=mc^2\:$ equation.
This equation states a relationship between matter and energy.
Einstein said matter and energy are the two sides of the same coin and are interconvertible.
Though this relationship is not that obvious at the macroscopic level, it well explains the interaction between atoms and smaller particles such as protons, neutrons, electrons, muons, etc.
The nucleus of an atom is made up of protons and neutrons.
Every atom has a particular binding energy that is required to break the nucleus into its constituent particles, and separate them up to a significant distance.
Equivalently, the binding energy is also the energy lost while combining protons and neutrons (collectively called nucleons) into a single nucleus (the opposite of the case above this).
Some energy is lost in the process of combining nucleons because the positive nuclei have to use a certain amount of energy to overcome the electrostatic repulsion amidst the nucleons up to a certain extent so that the strong nuclear force can come into play.
The strong nuclear force is stronger than the electrostatic force due to which, the nucleons (protons and neutrons) are successfully bonded with certain binding energy (lost in the process) which is compensated by a little loss of their mass.
Thus, we can say that the (binding) energy is lost in the form of mass. The reaction proceeds as follows –
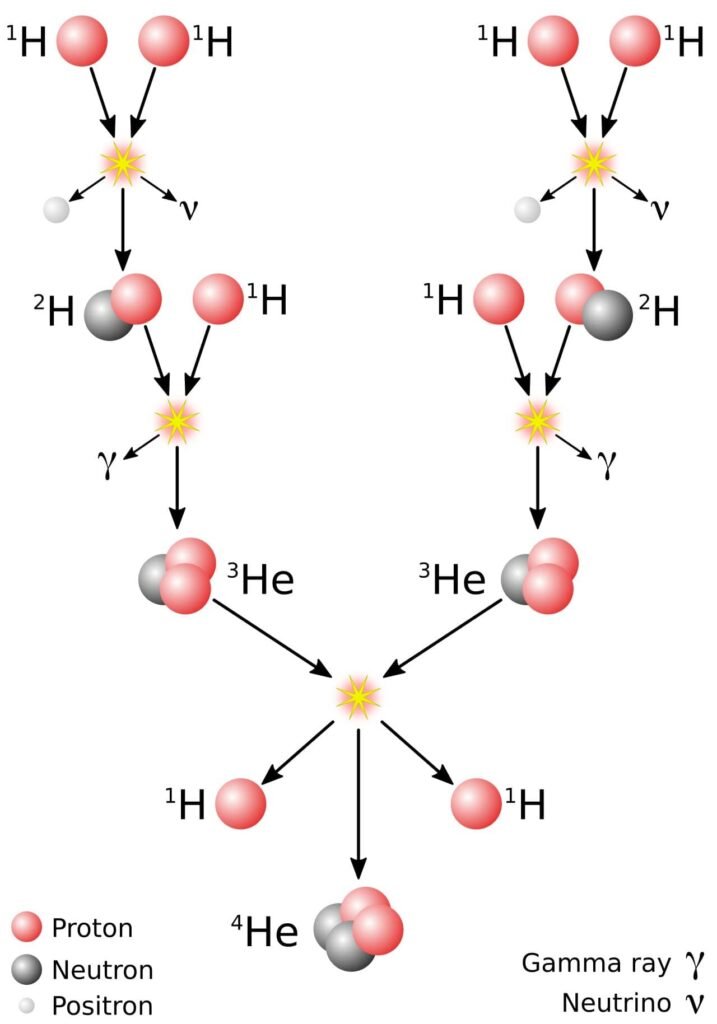
In a typical hydrogen fusion reaction, protons lose about 0.65% of their total mass. If we talk about a star like the Sun, it fuses almost 620 million tons of hydrogen every second.
Hence, the Sun loses almost 4 million tons of mass every second in the form of energy. Multiply it by c2 (9 × 1016) and you’ll get the energy (in joules) released by the Sun in one second.
An interesting thing to note down is that the fusion reaction releases energy in the form of the kinetic energy of alpha particles and neutrino, along with gamma radiation (an electromagnetic wave with very high energy).
But we don’t experience any gamma radiation from the Sun. If we did, then we would’ve been burned up already.
It is because most of its energy is absorbed during the journey of light from the core of the sun to its surface. Yes, that is a long trip indeed.
It takes about 100,000 years for light to reach from the sun’s core to its surface. Thereafter, light particles travel at their typical speed to reach Earth in just over 8 minutes.
Usually, ultraviolet, visible, and infrared radiation are the ones that make it to the star’s surface and then to the Earth. These radiations are of much lower energy, and also safer.
Structure of a Star
Different types of stars exist in the Universe and each of them has different structures.
However, broadly speaking, we can classify them as low-mass stars (dwarfs), main-sequence stars, giant stars, and super-giant stars.
The basic structure of a star looks like this –
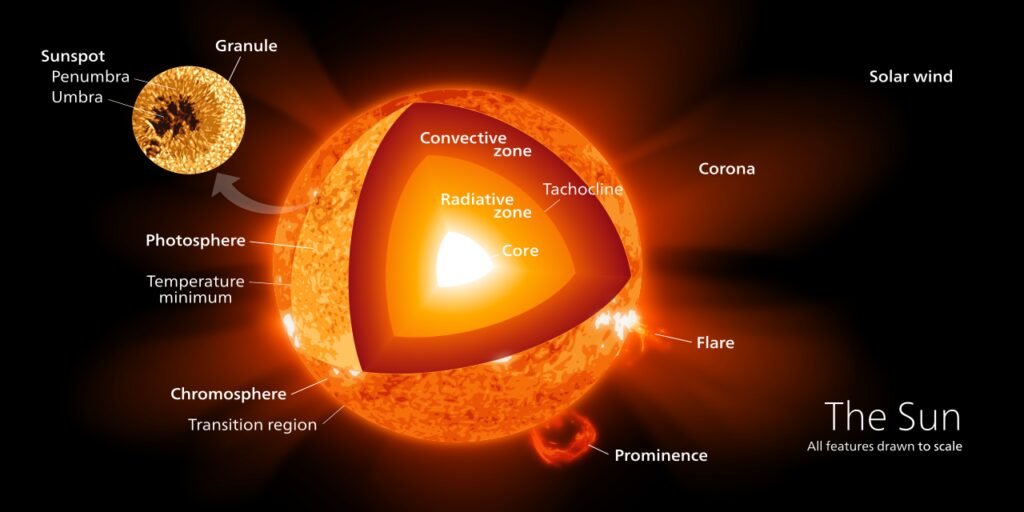
Core of a Star
It is the densest region of a star at its center, the temperature here is generally more than 10 million degrees celsius in main-sequence stars.
Such a high temperature is required to have fusion reactions in such dense conditions.
In massive stars, the core may have two divisions – outer core, and inner core. The core is also the heaviest part of a star as it has the highest density.
Radiation Zone
It transports the energy from the core to the outer layer of the star through radiation. Here temperature decreases from 15 million degrees celsius to 1.5 million degrees celsius.
It is about 75% hydrogen and 24% helium because most of the atoms here lack electrons, which can’t absorb photons for convection to the surface.
Most photons just bounce around, due to which, it takes hundreds of years for light to go across the radiation zone of a star, and this period is even longer in massive stars.
The location of the radiation zone also depends upon the star’s mass.
Stars with a mass of less than 1.2 solar masses, mostly have their radiation zone right above the core, but in massive stars, the region around the core and core itself becomes a convection zone and the radiation zone lies above it.
Convection Zone
It is the unstable zone of a star that is made up of plasma. In this region, the energy is transported through convection. Here, pressure and density gradually decrease.
The movement of plasma creates a circular vortex that rises, cools down as its buoyancy decreases, and then sinks.
It’s similar to what happens when we boil water. The water heats up at the bottom and rises while the upper (cold) layer sinks and heats on reaching the bottom, following the same cycle.
Photosphere
It is the thin outer shell of a star from which light is emitted.
99% of the light that we receive from the sun (and other stars) comes from the photosphere.
This layer is opaque to most of the wavelengths as the temperature is low enough for the plasma.
It is composed of convection cells or granules that, when looked at closely, are similar to the surface of boiling water.
Chromosphere
The chromosphere is the outermost layer that consists of the atmosphere of a star.
Sun’s chromosphere can’t be seen directly, but only during a total eclipse with specialized telescopes.
It is located about 10,000 kilometers above the photosphere and is reddish in color.
Its density is 10-8 times that of the earth’s atmosphere at sea level.
Most of the main sequence stars have the same structure, but low-mass stars such as red dwarfs and massive stars have slightly different structures.
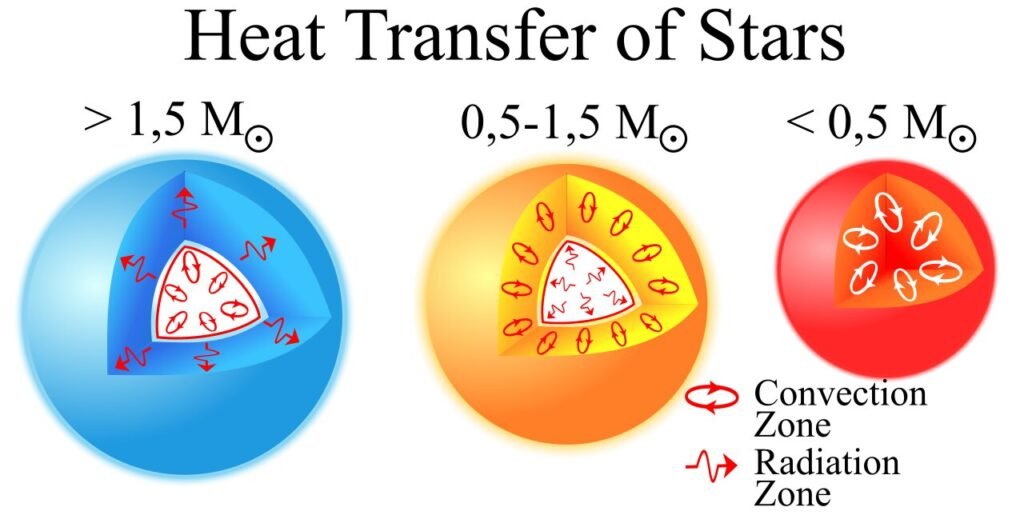
Different Types of Stars
Although stars can be classified into numerous categories based on numerous parameters, we’ll, herein, talk about some of the most common types of stars that are quite abundant in the universe.
Red Dwarfs
Red Dwarfs do not have a radiative zone. They consist of a dense core, a convection zone, and a photosphere.
Since the whole star is convective in nature, hydrogen all over the star is available for fusion.
Also, since the pressure at the center is not that intense, it fuses the hydrogen at a slower rate.
Due to this, red dwarfs have a very long lifespan; a trillion years sounds like a good approximation for that.
In stars like the Sun, or even heavier ones, the core is separated from the rest of the mass.
Due to this, they have lesser fuel to fuse and that, in turn, happens at a higher rate, thus, heavier stars have a shorter lifespan.
Yellow Dwarfs
Yellow Dwarfs are also called G-type main-sequence stars (or G stars, for short), these stars have a mass of about 0.9 to 1.1 solar masses.
It is, hence, self-explanatory that our Sun, too, is a yellow dwarf.
Main-sequence stars can be thought of as grown-up stars (which are past their childhood).
Yellow Dwarfs have a lifetime of about 10 to 12 billion years.
They are sustained by hydrogen fusing into helium at their core until they die as White Dwarfs.
White Dwarfs
White Dwarfs are highly dense stars having mass comparable to our Sun but have volume near to that of Earth.
Unlike yellow dwarfs and red dwarfs, white dwarfs are stellar remnants i.e. they are the leftovers of a dead star. No fusion takes place inside a white dwarf.
They are formed when average-sized stars, which are not big enough to eventually form neutron stars or black holes, undergo gravitational collapse.
White dwarfs have relatively low luminosity when compared to their parent stars.
Although inconsequential compared to neutron stars or black holes, white dwarfs are still quite dense.
They are capable of packing a mass equivalent to that of the Sun, in a volume equivalent to that of the earth.
Most of the white dwarfs have a mass of 0.5 to 0.7 solar masses. However, some could go as low as 0.17 solar masses, while some go as high as 1.3 solar masses.
While some white dwarfs can be involved in Type I supernovae, most of them cool down over a long period and eventually disappear into a black dwarf that no longer emits any heat or light.
Red Giants
Red Giants form when an average-sized star (or a yellow dwarf), similar to the Sun, runs out of its hydrogen fuel.
As the hydrogen-helium fusion stops, the core contracts, increasing the temperature of the star and causing an outburst of energy in the hydrogen shell.
This causes the star to expand enormously into a red giant, which is around millions or even billions of kilometers in diameter.
Also, this is what will happen to our Sun, in about 5 and a half billion years from now.
Click the link above to skip to the last section of our article that talks about the future and fate of our Sun (a future red giant) in detail.
Red Supergiants
Red Supergiants (elder siblings to the red giants) are among the biggest stars in the universe.
The structure of such high-mass stars is a bit more complex than that of our Sun.
They are formed when a star of about 10 solar masses runs out of hydrogen to fuse (into helium), and consequently swells up into a red supergiant.
The core temperature of a red supergiant can rise to around 500 million degrees celsius.
Such temperature is enough to fuse elements beyond helium and carbon.
Once hydrogen is out of the picture, different layers start forming inside the core as carbon fuses to make neon, magnesium, and sodium.
With the fusion of each element, the core contracts, and its temperature increases to fuse the heavier elements, this cycle continues all the way up to iron.
Eventually, the star fails at fusing iron and dies in a Type II supernova.
Fact: Stephenson 2-18 is the largest (red supergiant, or a possible red hypergiant) star in the universe. It has an approximate radius of 1.5 billion kilometers i.e. about the size of Saturn’s orbit.
Neutron Stars
Neutron stars are one of the weirdest astronomical objects in the universe.
They are only made up of neutrons and have an intense magnetic field, gravity, and density.
Just 20-25 kilometers across, neutron stars are so dense that one spoonful of a neutron star is as heavy as a whole city, while its gravity is billions of times more than that of the earth.
The first-ever neutron star was discovered in 1967, by Jocelyn Bell Burnell and Antony Hewish.
They developed a radio telescope to study the stars and galaxies. But they were interrupted by continuous radio signals, which were thought to be an error of the telescope itself.
It was later found that the signals were coming from a star PSR B1919+21 – an isolated, rotating neutron star. Young neutron stars rotate at the highest speeds.
This is because they generally retain the angular momentum of the star that they formed out of, but due to their small size, the retained angular momentum triggers a rapid rotation, which gradually slows down over time.
Some neutron stars also emit jets of electromagnetic radiation, which can be observed. These neutron stars are called pulsars.
Note: All pulsars are neutron stars, but not all neutron stars are pulsars.
Neutron stars are formed when massive stars run out of their stellar fuel and collapse under their weight.
The collapse is so strong that electrons surpass electron degeneracy pressure and fuse into the nucleus with protons, to form neutrons.
What’s left behind is a highly dense, compact, and rapidly spinning core of neutrons.
How do Stars Die? – What is a Supernova?
Supernova (plural supernovae) is basically the event that occurs upon the death of certain stars.
It is a ginormous cosmic explosion that occurs when the stars run out of their fuel i.e. when there are no lighter nuclei that can be fused into heavier ones.
The majority of the stars’ lives are used in fusing hydrogen nuclei into helium nuclei. This stage is called the main sequence.
After fusing all of the hydrogens into helium nuclei, the star then continues to fuse helium into carbon. However, the helium in the stellar core will eventually run out as well.
So to survive, a star must be hot enough to fuse heavier elements further, as the lighter ones become exhausted one by one.
Stars that are more than about 5 times the mass of the Sun (or 5 solar masses) can do this with ease.
They burn hydrogen, then helium, and then carbon, oxygen, silicon, neon, and so on… until they attempt to fuse iron (the super-heavy element).
Iron is a stable element that requires a lot of energy to fuse but releases no energy upon fusing.
With nothing left to counter the inward pull of the stellar core, the star implodes, blasting its outer layers into the universe; this happens rapidly.
Fact: A supernova can release more energy (in mere seconds) than the living star ever could, in its entire lifetime.
However, not all stars die so grandly.
Types of Supernovae
Supernovae are generally divided into two types:-
Type I
Type I supernovae are not the mainstream supernovae that we earlier read about. They are rather rarer, and different.
Type I supernovae occur in a binary star system (a pair of stars), which consists of a white dwarf and its companion star (a red giant or main-sequence star, or even another white dwarf).
The white dwarf (the remnant of a red giant) starts accreting (or stealing) matter from its companion star and grows in size.
As it grows in mass beyond the Chandrasekhar Limit (1.4 solar masses), it explodes, sending its remnant flying into space, and later vaporizes.
Type II
Type II supernovae occur in stars that are generally more than 10 times the mass of the sun.
These massive stars collapse at their core, blasting off their atmosphere, and form neutron stars.
Stars more massive than this collapse and form black holes, which are much denser than neutron stars.
Fact: SN 2016aps is the brightest supernova ever recorded. It was a type II supernova that was recorded in 2020, with an apparent magnitude of +18.11 (while the Sun has an apparent magnitude of -26.7).
Our Sun As a Star
Sun is the closest star that we can see from Earth, but this isn’t the only reason for our fascination with it. Sun is very special to us for a ton of reasons.
Sun has enabled life to exist on the earth amidst the hostile conditions of the dark cosmos.
Sun is categorized as a GV2-type main-sequence star which falls under the category of yellow dwarf stars.
Although astronomers may categorize the Sun as a low-mass star, the Sun is still brighter than 85% of the stars in our galaxy.
This is because most of the stars in our galaxy are red dwarfs, which have very low masses.
Most people think that our Sun is yellow in color, but it’s actually white.
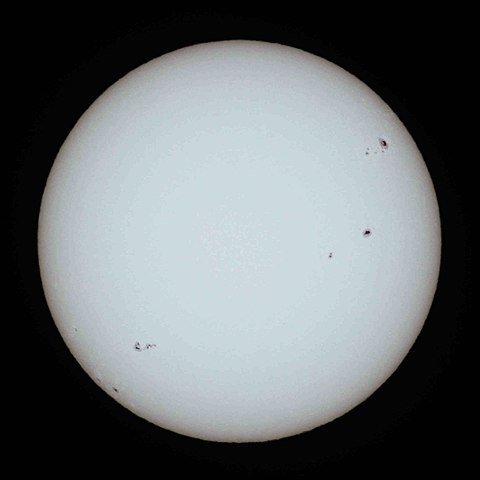
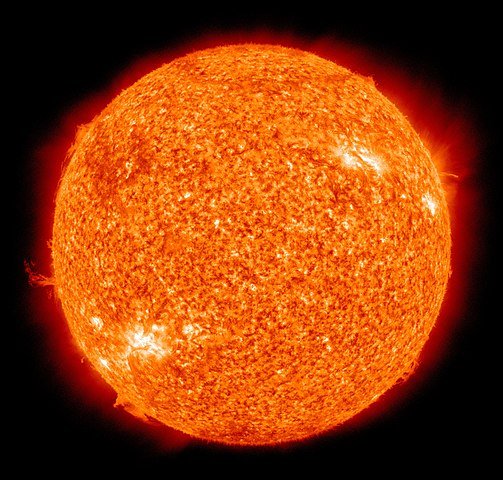
The exact mass of our sun is around 2 × 1030 kg which is almost equal to the mass of 330,000 piles of the Earth.
Sun constitutes over 99.8% of the mass of the solar system, most of its mass is due to hydrogen (73%) and helium (25%).
It fuses almost 600 million tons of hydrogen every second in its core, releasing 4 million tons of mass in the form of energy (heat and radiation), which is equivalent to 4 × 1023 kilowatts of power.
The sun has a diameter of about 1.4 million kilometers, which is about 109 times that of Earth.
Ancient people understood the significance of the Sun in sustaining life on Earth, which is why almost all ancient civilizations considered the Sun a Deity or God.
On an average cloudless day, we obtain almost 1000w/m2 worth of power on Earth, from the Sun.
The total radiation that we receive from the sun, consists of 50% infrared, 40% visible light, and 10% UV rays, which mostly get filtered through the ozone layer of the Earth.
What Will Happen To Our Sun?
Our Sun is almost 4.6 billion years old and will thrive for around 5.4 billion years more.
During its last stage of life, it will exit the main-sequence stage of its lifetime.
With its hydrogen exhausted in the core, the inert helium ash that has built up there will become unstable and collapse under its own weight.
This will cause the core to heat up and get denser, causing the overall Sun to grow in size and enter the Red Giant phase of its evolution.
It is calculated that the expanding Sun will grow large enough to engulf the orbits of Mercury, Venus, and maybe even Earth.
Thereafter, the Sun will continue fusing helium into heavier elements like carbon and oxygen.
The Red Giant phase is a quite unstable phase that causes the star(s) to collapse & re-inflate in repeated cycles.
With each cycle of contraction and inflation, the Sun will begin spewing its dust & gasses into its surroundings, and the Sun’s atmosphere will slowly dissipate over the years to form a planetary nebula.
With no helium left to fuse (and incapable of fusing carbon and oxygen into heavier elements), the Sun will eventually be left with a compact, white-hot core of carbon and oxygen.
The Sun now becomes a White Dwarf (the final stage of the Sun).
The White Dwarf sits there like a hot flashlight, illuminating its surroundings (that mostly comprise remnants of the Red Giant), until it cools down and silently evaporates into the sheer silence of the cosmos, in about 10,000 years.
Note that this is not a fate that is met by all the stars, this is just what happens to average-sized stars or yellow dwarfs like our Sun.
Stars heavier than our Sun traverse a different pathway of life and transform not into hot little white dwarfs, but ferocious neutron stars or even monstrous black holes (go to What is a Supernova in this article, for more info).
By that time, humanity would have either left the planet long ago or would’ve already met its extinction.
So until then, as the Roman philosopher, Marcus Aurelius said, “Dwell on the beauty of life. Watch the stars, and see yourself running with them.”
As we contemplate these large celestial bodies, we can’t help but shiver in both ecstasy and horror of the infinite cosmos.
So, after having learned so much about stars, it would now be more reasonable to sing:
Fuse fuse humongous stars, now I do know what you are!