Black holes are the most mysterious entities existing in the universe and are glorified as dark regions in space-time sucking in matter and energy.
Let’s have deeper insights into a black hole. Its name unequivocally explains it all.
A black hole in general consists of a hole at its center and a surrounding black part.
The hole is the central point of a black hole where the singularity resides, and the black part surrounds the singularity and is the only part we can somehow manage to observe.
There is a boundary between these two parts, and Astronomers call it the event horizon – no one knows what happens beyond this boundary.

Black Holes have a really dominant gravitational influence, which is such intense that even light – the fastest entity in the universe – cannot escape.
That’s also the black holes are black, because we see objects when light hits an object and then reaches our eyes.
The gravity and density at the singularity are infinite because all of the mass had been squeezed into a dimensionless point.
This particular region tears apart any physics we know.
The physics of singularity is really exotic and remains to us until now because conventional mathematics doesn’t seem to work here.
So, that’s how black holes are currently the weirdest thing (except quantum mechanics) to exist in the universe in the knowledge of Humanity.
How is a Black Hole Formed?
Basically, a black hole is formed when a massive star dies.
A star on the verge of its death gravitationally collapses onto its center.
To understand better, we need to know what happens when a star dies.
Star shines, or one must say produce energy which the help of fusion reactions going inside its core.
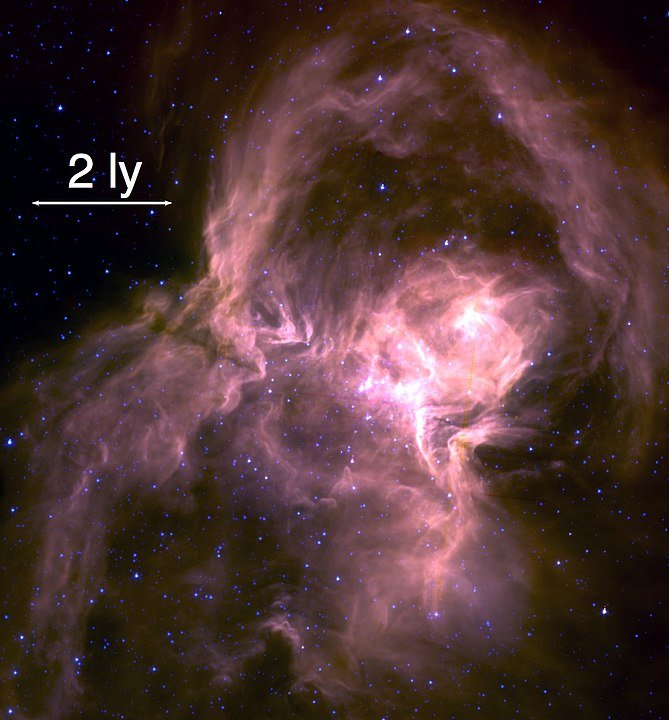
There is plenty of Hydrogen in the star, which is constantly fused to form Helium, these Helium atoms fuse to form Lithium, and the process continues till the formation of Iron.
The nuclei of Iron atoms are the most stable nuclei in the periodic table.
Hence further fusion will lead to instability, and hence the fusion reaction stops after the formation of the iron.
Now, since no fusion reaction occurs inside the star, there is no pressure inside to counter its gravity, and it starts collapsing, leading to energetic supernovas.
Depending on the mass of the star, there are three possible outcomes of this event –
If the star’s mass is less than 1.5 times the mass of the sun(solar mass), then the star would collapse into a white dwarf, which consists of electrons and degenerate matter (degenerate matter is the atoms that lost their electrons due to extreme temperature and pressure.
White dwarfs are dense, so dense that an Earth-sized white dwarf can be as heavy as the Sun.
Fun fact – the heavier the degenerate matter, the smaller the size of a white dwarf.
If the star’s mass is between 1.5 to 3.3 times the solar mass, it will collapse into a neutron star.
The Force of gravity is so strong that electrons are ripped off the atoms and combined with a proton to give rise to neutrons.
So, a neutron star is mostly made up of neutrons with an ocean of electrons over the surface.
The internal structure of the neutron star is still unknown, but it is said that it is composed of a gluon-plasma soup.
If the mass of the star is above 3.3 times the solar mass, it becomes a black hole.
How do we know, what we know about Black Holes? – History
The concept of a black hole was first proposed around 1784, and it was Albert Einstein’s 1915 theory of general relativity that gave life to this idea.
The history of Black holes dates back to 1784 when an English clergyman John Michell published a paper describing a star that is so massive that its gravity doesn’t allow the light to go past around it.

The star’s density was similar to the sun’s, but its size should be greater by a factor of 500.
At that time, the theory of light was not well established, and there were arguments over the speed of light.
When the ‘theory of corpuscles‘ was dumped and the wave theory of light occupied its place, there was no way gravity could influence light, hence, John Michell’s theory was discarded.
In 1915, Einstein’s relativity indicated that Gravity does indeed influences the motion of light.
A black hole is formed due to the collapsing massive star on the verge of its death.
Initially, Physicists didn’t believe that such a bizarre thing could exist in reality.
Albert Einstein, whose General relativity predicted the existence of the Black Hole, himself didn’t believe that such a thing would ever exist.
He thought that due to the conservation of angular momentum, a point in time would come when centrifugal force would be enough to counter the effect of gravity and stop the collapsing star at some radius.
After a few months of discovering General Relativity, Karl Schwarzschild worked out a solution to Einstein’s General Theory of Relativity which describes the gravitational field of a point mass.
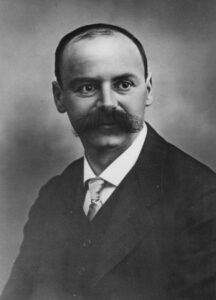
In 1931, Subrahmanyan Chandrasekhar demonstrated that Black holes could exist.
Using General Relativity, he concluded that a star with a mass higher than 1.4 times the solar mass would keep squeezing until it becomes a black hole called the Chandrashekhar limit.
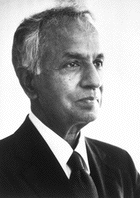
Many physicists opposed his theory, including Eddington and Lev Landau, because they believed there should be some unknown force that would stop the star from collapsing.
In their opinion, our universe cannot hold something which has any infinite value (black holes have infinite density and gravity at their center).
Later in 1939, Robert Oppenheimer proved that stars don’t collapse under the Chandrashekhar limit.
An under this limit becomes a neutron star that does not collapse further because of neutron degeneracy pressure.
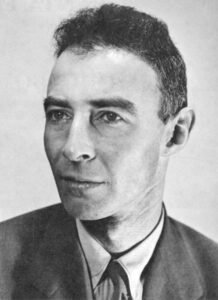
Still, there is a limit above which even a neutron star collapses and forms a Black Hole similarly as explained by Subrahmanyan Chandrasekhar earlier.
Though physicists could develop such great theories and predict neutron stars, black holes, pulsars, and whatnot, these bodies existed just in theories.
None of them were discovered until 1967 when the first pulsar was detected.
It was an indication to the Physicists that they were going in the right direction.
When Einstein showed that gravity could bend the path of light just as it bends the path of any other moving object – the reason we don’t observe this effect in our daily lives is that light moves really fast, and gravity pulls weak.
This was later confirmed by observations (Solar Eclipse of 29 May 1919; Light Bending Experiment 1917-1919), and the idea of black holes got revived.
A sufficiently massive body’s gravitational pull should be strong enough to not only bend light’s path but also keep it from escaping.
Facts about Black Holes
Black Holes are often called the vacuum cleaners of the universe, but it’s not a correct analogy for a black hole.
If we place a black hole of similar mass in the place of our Sun, planets will still revolve around it as usual.
A black hole imprints its effect only when we are too close to it.
Why is it so? to answer this particular question, we need to understand the Escape velocity of an object (If you’re already aware of it, you can skip this part).
Escape Velocity & Massive Objects
The escape velocity of an object is the velocity at which any particle on the surface of a gravitationally dominant object can escape its gravitational influence.
The escape velocity of an object depends upon its mass and size.
It can be represented in the form of the expression below:
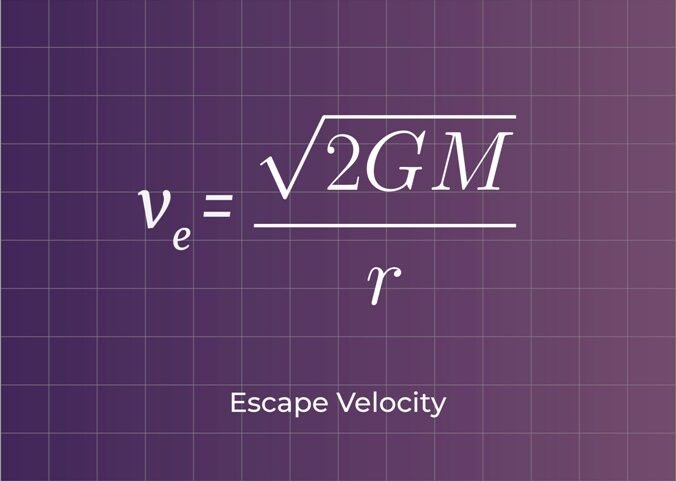
The escape velocity of our earth is 11.2 km/s, and the sun has an escape velocity of around 600 km/s.
The escape velocity of a neutron star is around 150,000km/s, which is just half the speed of light.
Now, if we squeeze an even more massive object, its escape velocity will go beyond the speed of light.
So, we can say that the event horizon of a black hole is the surface where the escape velocity for any object will be equal to the speed of light.
First-ever image of a Black Hole
Around April 2017, The European Southern Observatory(ESA) announced that they have some big news to share.
Later on 10th April 2017, The global collaboration – Event Horizon Telescope (EHT) project, shared the first-ever images of a black hole’s event horizon.
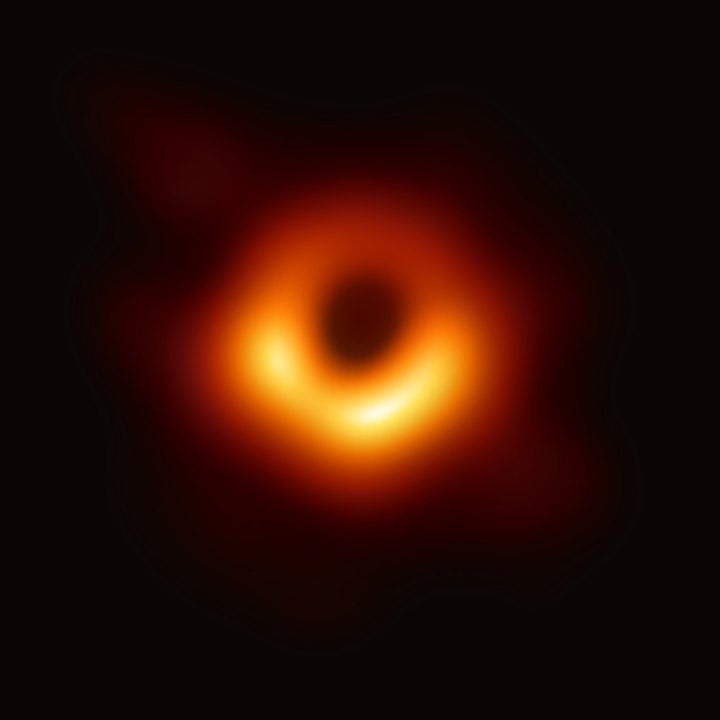
A great round of applause, and hats off to every who was working out the project.
This project wouldn’t be successful without mutual hard work, cooperation, and global collaboration.
A technique called Very-long-baseline interferometry(VLBI) was used to capture the black hole’s image.
VLBI works by creating an array of smaller telescopes that can be synchronized to focus on the same object simultaneously, eventually acting as a giant virtual telescope.
This technique has been used to track spacecraft and capture images of distant cosmic radio sources, such as quasars.
To test VLBI for imaging a black hole and several computer algorithms for sorting and synchronizing data, the Event Horizon Telescope team decided on two targets, each offering unique challenges.
The closest supermassive black hole to Earth is Sagittarius A* (an asterisk is an astronomical standard for denoting a black hole) in our Milky Way galaxy.
It interested the team because it is in our own galaxy – 26,000 light-years (156 quadrillion miles) away.
However, it’s not the only black hole in our galaxy but is the one that appears largest from Earth.
The team faced problems in producing its images earlier due to the ‘pollution,’ which means they had to look through this pollution caused by stars and dust to image it.
Eventually, there would be more data to filter out when processing the image.
Since Sagittarius A* is near and relatively larger, the EHT team chose it as one of its two targets.
Later, the team was finally able to produce the image of Sagittarius A* which was released on 12th May 2022.
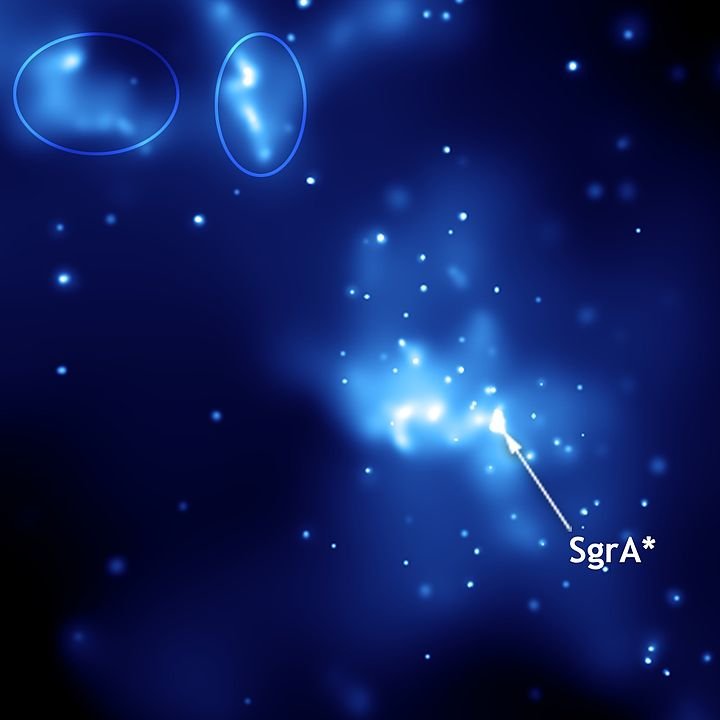
The second target was the supermassive black hole M87*.
One of the largest known supermassive black holes, M87*, is located at the center of the gargantuan elliptical galaxy Messier 87, or M87, 53 million light-years (318 quintillion miles) away.
Even more massive than Sagittarius A*, which contains 4 million solar masses, M87* contains 6.5 billion solar masses.
One solar mass is equivalent to the mass of our Sun, approximately 2×10³⁰ kilograms.
In addition to its size, M87* interested scientists because, unlike Sagittarius A*, it is an active black hole, with matter falling into it and spewing out in the form of jets of particles that are accelerated to velocities near the speed of light.
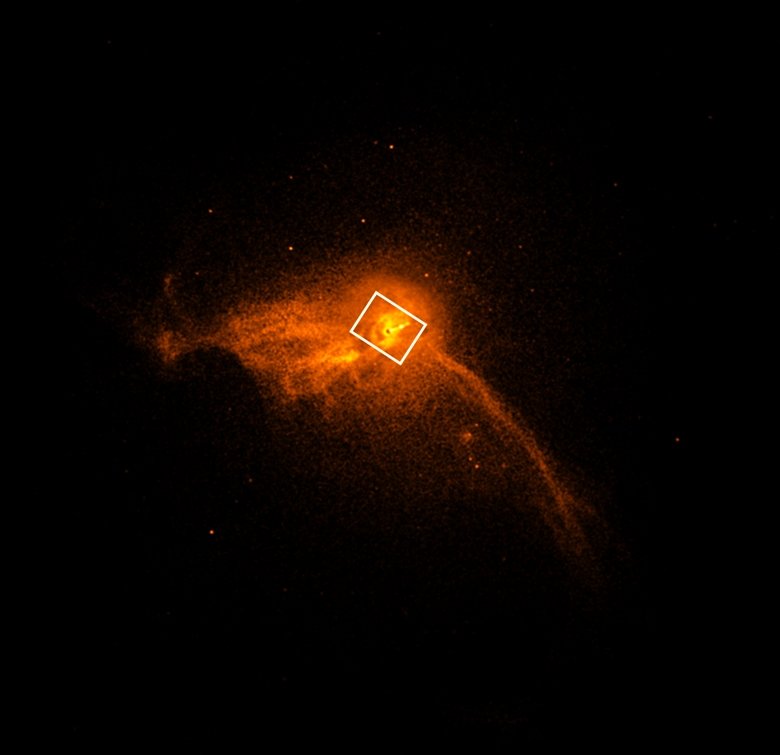
The distance of M87* makes capturing its image more of a challenge than the relatively nearer Sagittarius A*.
As described by Katie Bouman, a computer scientist with the EHT who led the development of one of the algorithms used to sort telescope data during the processing of the historical image.
It’s pretty much similar to capturing an image of an orange on the surface of the Moon.
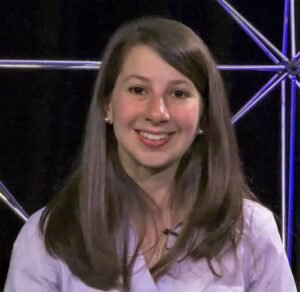
Surpassing all challenges, the EHT project team was finally able to capture the image of the supermassive black hole in the galaxy Messier 87(M87).
Later, ESA arranged a press conference on 10th April 2017, where they revealed this historic breakthrough in Astronomy.
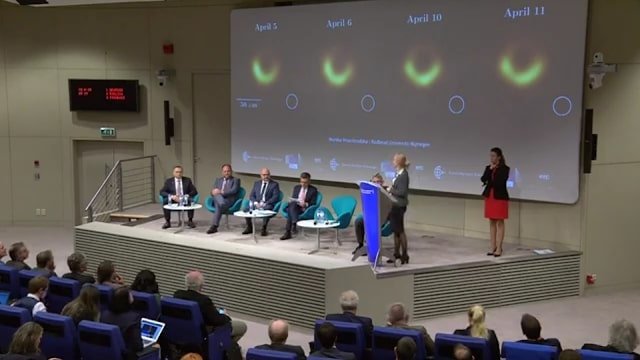
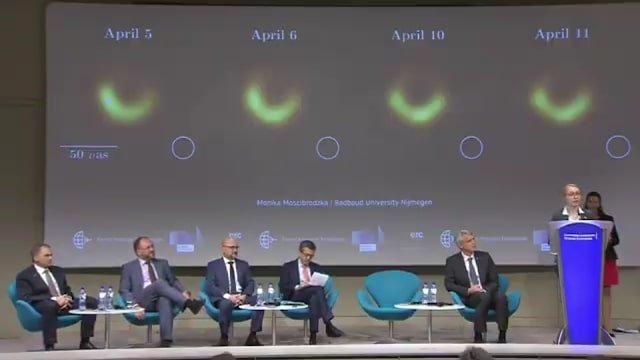
The ring-like structure around the black hole is called the “Ring of Fire“.
It is the matter around the hole and the black circle is the part from where light cannot escape and reach us.
Brightness is bolder at the bottom which tells that the matter which is being sucked into it and along with the black hole is rotating, the spin is observed to be clockwise.
Properties & Types of Black Holes
Just like any other physical entity in the universe, black holes also possess certain physical properties:
How big is a black hole?
Did you know?
The largest black hole ever found in the universe has a diameter of about 78 billion miles, which is about 40% the size of our solar system and the smallest black hole ever found is about 15 miles in diameter.
Primordial Black Holes
These are the hypothetical black holes that were formed due to heterogeneous conditions created just after the Big Bang.
Their mass is smaller than that of the Earth and their size could even be smaller than an atom because of their smaller size, they are nearly impossible to detect.
Stellar Mass Black Holes
These are the most widespread blackholes, their mass lies within a range of 5 to 25 solar masses but their size could be similar to that of a small city (around 10 miles across).
Intermediate Mass Black Holes (IMBH)
It is the class of black holes with a mass within the range of 10^2 -10^5 times the solar mass.
Till now, not a lot of them have been discovered so far.
Supermassive Black Holes (SMBH)
As the name suggests, these black holes are gigantic on the Astronomical Scale.
They are at least a million if not a billion times the solar mass.
Some of them even weigh more than an entire galaxy.
Currently, Tonantzintla 618 is the biggest black hole we have ever discovered.
Its mass is around 66 billion times the solar mass, these types of black holes are generally located at the center of every galaxy.
No-hair conjecture
According to no-hair conjecture, no matter what goes inside the black hole, it will always have three independent fundamental physical properties:
- Charge
- Mass
- Angular momentum
It is believed that the black hole can’t have any other features but still, there is no solution for it.
These are the only values we can obtain while being outside the black hole and we can measure them.
Physicists have classified black holes based on the above properties.
Classification of Black holes based on Charge & Angular Momentum
Black holes can be classified according to their charge and angular momentum.
A black hole that possesses some electric charge is called a charged black hole.
As the electromagnetic repulsion in compressing an electrically charged mass is much greater than the gravitational attraction (by about 40 orders of magnitude), it is not expected that black holes with a significant electric charge will be formed in nature.
Schwarzschild Black Hole
These types of Black holes don’t have any charge or angular momentum.
These types of black holes are also called ‘Static Black Holes’ and mass is its only significant property.
It is predicted on the basis of the Schwarzschild metric which is the solution of General Relativity for a Black Hole.
Kerr Black Hole
These black holes have angular momentum but lack any kind of charge in them.
They are described by using Kerr metric or Kerr geometry which is just an extension of the Schwarzschild metric to the rotating bodies.
Reissner–Nordström Black Hole
These are charged, non-rotating Black Holes.
They are described by the Reissner–Nordström metric which is the solution of Einstein-Maxwell equations.
Kerr-Newman Black Hole
Charged rotating black holes are known as Kerr – Newman Black Holes which are described by Kerr-Newman Black Hole
Unsolved problems of Black Holes
It’s already clear that we don’t know a lot of things about these exotic black holes.
They happen to possess a ‘singularity’ when all the physics we know, gets crushed.
Here are two concerning questions that scientists are seeking a solution for:
The Information Paradox
According to quantum mechanics, Information can never be created or destroyed is called the law of conservation of information but this law seems to get messed up around a black hole.
Is physical information lost in a black hole?
After an object is engulfed by a Black Hole, we can never retrieve it back again. Now, the problem is – what happened to the quantum information(configuration and nature of its particles) of that object?
This paradox exists because of inconsistencies between Quantum Mechanics and the General Theory of Relativity.
Entropy & Thermodynamics
In 1971, Prof. Stephen Hawking demonstrated under general conditions that the total area of the event horizons of any collection of classical black holes could never decrease, even if they collide and merge.
This result is termed the second law of black hole mechanics.
It is seemingly similar to the second law of thermodynamics, which states that the total entropy of an isolated system cannot decrease.
Classical black holes were assumed to possess absolute zero temperature and zero entropy.
If this were the case, the second law of thermodynamics would be violated.
When matter having a certain entropy would enter a black hole, there must be a resulting decrease in the total entropy of the universe, as the black hole has to have zero entropy.
Connections with the laws of thermodynamics were further motivated by Hawking’s discovery that quantum field theory predicts that a black hole radiates blackbody radiation at a constant temperature.
This causes a violation of the second law of black hole mechanics (as mentioned above, the total area of the event horizons of any collection of classical black holes can never decrease) because the radiation will take away energy from the black hole, causing it to shrink.
A confusing feature is that the entropy of a black hole depends upon its area rather than on its volume because entropy is generally an extensive quantity that depends linearly on the volume of the system.
To back this up, Gerard ‘t Hooft and Leonard Susskind proposed the holographic principle, which suggests – anything that happens in a volume of space-time can be described by data on the boundary of that volume.
In statistical physics, we define entropy as counting the number of microscopic configurations of a system with the same macroscopic qualities (such as mass, charge, pressure, etc.)
Generally, gravity is weak, but at black holes, it gets profound enough to mess things up at the microscopic level, and that’s why without a satisfactory theory of quantum gravity, one cannot perform any calculations for the entropy of black holes.