The biological world is always undergoing significant constant changes brought down by Homo sapiens.
Since the appearance of life on Earth, all life has advanced to evolve as per the Darwinian evolutionary theory, organisms developed by a series of random mutations and genetic variations.
Those mutations and variations were sometimes for the advantage of species and sometimes they were not, not every mutation is good. So mutations for the betterment of species helped an organism reproduce and survive in adverse conditions.
We wish for a genome that is perfect in every way, free from undesirable mutations and errors in the sequence of the DNA.
The development of recombinant DNA technology in the 70s embarked on a new age in the field of molecular biology.
Even we homo-sapiens went through drastic changes; of course, humans didn’t always look like this as we do now, and frankly, humans have been at the mercy of genetic changes for shaping our current form, until now.
Scientists have now developed tools to fully regulate this primal process of modifying genes and one of those tools is CRISPR.
With genetic engineering, we will be able to increase the complexity of our DNA, and improve the human race. But it will be a slow process because one will have to wait about 18 years to see the effects of changes on the genetic code.
Stephen Hawking, Theoretical Physicist
We’ve certainly come a long way since Stephen Hawking said this back in 2001, gene editing has gotten better but there’s still a long way to go.
CRISPR gene editing has brought down revolutionary changes in the field of biotechnology by allowing scientists to tweak the genome in living being as per their desire.
It started as a bacterial immune system and is now a promising way to permanently modify not only the human genome but modify every single species on Earth according to our needs.
What is Gene/Genome Editing?
Gene editing also known as genome editing comprises ways to give scientists the ability to edit the genes of an organism of the organism, these changes in the DNA result in modification of physical traits, like changes in eye color, hair color or so.
These techniques act like molecular scissors that cut the DNA at specific locations, by which scientists can add, remove and alter the DNA at those locations.
One of the first gene editing techniques was developed in the late 1990s.
As of the present scenario, gene editing has thrived for a broad range of experiments ranging from plants to animals.
What is ‘CRISPR’ Gene Editing?
CRISPR stands for “clustered regularly interspaced short palindromic repeats”, it is one of many ways by which gene editing can be done and CRISPR is faster, cheaper, and better than the older technologies, *simple but effective*.
CRISPR was originally derived from a naturally occurring bacterial immune defense system and it can be used to edit, program, and modify genes in living organisms. It was first discovered in archaea and later in bacteria by Spanish scientist Francisco Mojica in 1993.
What is Archaea?
Single-celled microorganisms with “structure” similar to bacteria but in terms of evolution, they lie different.
Francisco suggested that CRISPRs are a part of the bacterial defense system against bacteriophages. [1]
The bacterial DNA consists of spacer sequences (spacer sequence is the non-coding region of DNA), and fragments of the genetic code of past invaders, this system develops a sort of memory to detect and destroy invaders when they attack again.
The bacteria use Cas9 or similar enzymes to cut off the DNA, which disables the virus.
In genome engineering CRISPR or CRISPR-Cas9 is used to loosely refer to other various CRISPR and other systems.
Francisco’s theory was later proved experimentally by Phillipe Horvath and his team in 2007.
In January 2013, Zhang Lab successfully edited the genome of the eukaryotic cells (Human and mouse cells), they showed that the system could be programmed to target multiple loci on the genome.
Who Discovered CRISPR-Cas9?
The 2020 Nobel prize for Chemistry was awarded to Jennifer Doudna and Emmanuelle Charpentier for their groundbreaking discovery of one the most advanced tools we have for gene editing “CRISPR-Cas9“, also abbreviated as “molecular scissors“.
Charpentier published her work on CRISPR in 2011 and in the same year, she made a collaboration with an American Biochemist Jennifer Doudna, together they worked on simplifying the molecular mechanisms of CRISPR to make them easy to use.
Charpentier and Doudna proved that these molecular scissors are programmable and they can cut DNA at any prescribed site.
How Does CRISPR Gene Editing Work?
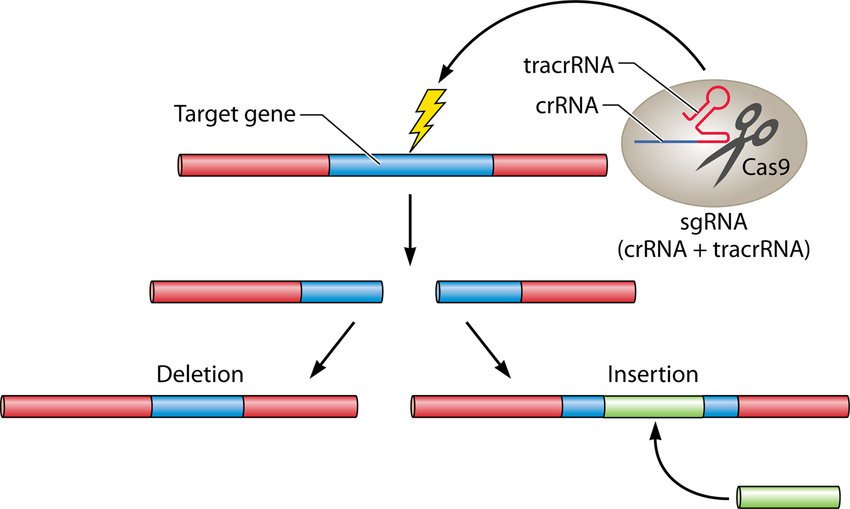
Researchers use a guide RNA sequence that binds to a specific site in the cell’s DNA.
This guide RNA which was introduced earlier to the cell also binds to the Cas9 enzyme, guide RNA then identifies the targeted DNA sequence and uses the Cas9 enzyme to cut the required section. [2]
Once the DNA is cut, researchers use the cell’s repair machinery to add or remove DNA pieces. CRISPR is an efficient tool to customize and edit the 3 billion base pairs in the human genome.
CRISPR-Cas is itself capable of cutting the DNA, so needn’t be paired with other cleaving enzymes. [3]
CRISPR-Cas9 can be used to target multiple genes at a time, which makes this technology stand apart from all the other gene editing technologies.
CRISPR gene editing is broadly classified into two classes, class I and class II.
The major difference between class I and class II is the effector molecules, while class I effectors contain multiple subunits, class II are single large proteins.
Those systems are distinguishable by the virtue of unique genes:
- Cas3, class I
- Cas9, class II
- Cas12/Cpf1, class II
Class, I systems can be found in most bacteria and archaea and can target both DNA and RNA.
Class II systems aren’t as common as class I, those systems are found only in bacteria, those systems and can target both DNA and RNA depending upon the type.
Usually, class II systems are used to cleave DNA at sites because of their easy preparation and it’s cheaper.
Cas9 is the most commonly used enzyme in research and gene editing.
CRISPR-Cas9 Gene Editing
Cas9 stands for CRISPR-associated protein 9, it is a dual RNA-guided endonuclease, associated with CRISPR it cannot just cut any DNA it needs Protospacer Adjacent Motifs (PAM).
PAM is essentially a targeting component that distinguishes self-DNA from non-self-DNA.
Cas9 enzyme cuts the DNA at two sites because it is dual-guided, when Cas9 cuts the DNA it leaves blunt ends.
Using Cas9 enzyme to make cuts is somewhat like tearing paper, non-precise, and kind of tainted.
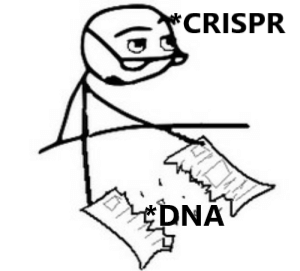
And the blunt ends in the DNA is something no one would want, because when those blunt ends are joined back it results in mutations, and if those mutations are in germ cells.
Phew, you need to be scared of what happens next because that mutation could result in something which has never been seen before in the entire medical history.
So the cuts need to be precise and on the exact locations where the DNA needs to be sliced down.
Now, we introduce CRISPR-Cpf1, which is relatively a better and cheaper version of CRISPR-Cas9.
CRISPR-Cas12 (Cpf1) Gene Editing
CRISPR-Cpf1 is an RNA-guided endonuclease analogous to CRISPR-Cas9 but shows unique features[4].
Cpf1 stands for CRISPR from Prevotella and Francisella1, previously known as Cas12.
Prevotella and Francisella1 are the bacteria from which CRISPR-CPF1 is derived.
Cpf1 has been acclaimed as a better substitute for CRISPR-Cas9 because even cutting off the DNA there is always a scope for re-editing the DNA.
When Cpf1 cuts the DNA it’s far from the recognition site, meaning that if the gene on site is mutated it can be edited again to achieve the right edit.
The size of Cpf1 is relatively smaller than Cas9 which makes it easier to deliver in cells and tissues.
Multiple recognition sites can be targeted while working with Cpf1, unlike Cas9.
Cpf1 is a relatively newer technology to be harnessed and used because of its feasibility but it is not as famous as Cas9.
CRISPR-Cas9 Germline Editing
With technologies like CRISPR, editing of somatic and germ cells is becoming feasible.
Germline editing is associated with germ cells, germ cells are the reproductive cells in the body, for females, it is an egg cell or ova and for males, it is sperm.
Genome editing has been shown to work in mammalian embryos, such as monkeys, mice, and rats.
Technologies like CRISPR-Cas9 are used to edit the embryo and the edited embryos can be implanted into foster parents (animals), when born, those individuals carry precise changes in their DNA.
Human embryos are quite similar to those embryos, so this implies a possibility that one may be able to edit the human embryo.
In 2015 a study was published which showed that CRISPR-Cas9 can be used for human germline editing, however, the embryos developed for the experiment weren’t capable of development for the first time ever[5].
Benefits of CRISPR Gene Editing
The development of gene editing technologies enables gene modification in sorts of living beings, these techniques can also be used to customize human characteristics for therapeutic enhancements.
Gene editing has a wide range of benefits ranging from the treatment of various diseases to the development of transgenic foods.
Gene editing is also presented to treat infertility, one possible application is NOA (non-obstructive azoospermia) in males.
Scientists are trying to “De-extinct” the Woolly Mammoth, using gene editing techniques.
Colossal Biosciences, a biotechnology company aims to genetically resurrect the woolly mammoth by combining its genes with the Asian elephant’s DNA [6].
So, maybe there’s a chance that we get dinosaurs back :p, rooting for the stegosaurus fam!
CRISPR holds enormous potential to improve our world and ameliorate the genetic makeup of the organisms in our ecosystem.
The myriad prospective CRISPR-Cas products have the ability to solve many of our 21st-century world’s problems.
CRISPR gene editing has brought drastic changes in the biological world by making it possible for scientists to modify organisms according to their needs.
CRISPR has the potential to be used for treating genetic diseases, curing blindness, and even humanizing animals.
Scientists can develop drought-resistant crops, mosquitos that do not transmit malaria, tomatoes that do not rot, and apples that do not oxidize (turn brown when cut).
Any problem, ranging from medical and health issues to food and agricultural production, all of this in the blink of an eye (it will take longer :p), made possible by the molecular scissors of all species.
CRISPR Human Genome Editing
CRISPR gene editing can be used on humans because of its extraordinary ability to snip DNA at any location. Its ability to cut DNA at locations makes it a potential tool to fix genetic mutations in the DNA.
Early research in human models suggests that CRISPR can be used on an array of diseases ranging from cancer to genetic diseases like thalassemia.
Early clinical trials to cure sickle cell anemia and thalassemia in humans have some very promising results[7].
That ensures a possibility that genetic disorders and disabilities may have a cure using CRISPR.
Human Genome editing with CRISPR opens up a world of possibilities for enhancing human health and wellness by allowing us to alter our genetic code through gene editing
Ethical Concerns With CRISPR Germline Editing
CRISPR is godlike, there are endless possibilities to modify anything about anyone at any hour.
Possibilities are endless no doubt, but as soon as it starts to deviate from noble purposes to self-sufficing ones, things(…) are going to get out of hand.
So when working with CRISPR, there should be certain regulations that inhibit us from going bananas on genome editing.
The ethical implications of CRISPR gene editing have been a major concern with this technology.
Off-target mutations and mosaicism are one of the major drawbacks of CRISPR-Cas9, and it’s a drawback that can’t be ignored easily.
Mosaicism occurs when a person has two or more genetically different sets of cells in their body. Down’s syndrome is an example of mosaicism.
Germ cells are reproducing cells in the body meaning that every time a body reproduces those cells are going to pass down genetic information to the offspring.
Whatever genetic changes are done in germ cells in the individual there is always a fair chance that those mutations will be passed down to the offspring[8].
If the editing fails a whole bunch of ethical, moral, and legal issues would arise.
Additionally, there may be moral concerns about who should decide which modifications are acceptable and how these changes may affect future generations.
Some countries have banned or restricted the use of genetic modification techniques on food products and animals, while others have imposed strict labeling requirements on genetically modified organisms (GMOs).
There are certain rules and regulations while using genome editing, for instance, there is a complete ban on germline editing by federal laws in the United States.
However, there is no prohibition on germline editing that is carried out through private funding but to sell the product in the US, you need to get FDA approval.
And there is a ban on the genetic manipulation of human embryos in many countries.
CRISPR-Cas9 is efficient enough to be used on our species but the concern is WHAT IF IT FAILS?
What if CRISPR Germline Editing Fails?
Well, failure in this case means a lifetime mess, one’s future generations are potential candidates for, quite frankly, a messed up DNA set/genome.
And the individual is exposed to a ton of health-related problems whether it’s related to the heart, brain, or making a person more prone to cancer.
The magnitude of potential risks related to germline isn’t fully determined and to be honest not even completely known.
So there are both national and international policies to regulate embryo research and early interventions that apply to human genome/germline editing.
Basically, these policies are frameworks of the broad underlying ethical context of using humans as subjects for carrying out research on genomics, in this context germline editing.
The impact of germline editing on a societal level is concerned with eugenics, social justice, and equal access to gene editing technologies.
What is Eugenics?
Eugenics is an erroneous and immoral scientific theory of “racial improvement” and “planned breeding”, which beliefs in the fact that the human race can be perfected and so-called social ills can be eliminated through genetics and heredity.
The bioethical community in fact expresses specific concerns about this “enhancement” of genes that go beyond the treatment of medical disorders.
What if CRISPR Germline Editing Succeeds?
The technology is similar to weapons and drugs, immoral use such as the creation of a super baby should be banned forever.
Yang Hui, Shanghai Institute for Biological Sciences
Although, the potential risks with germline editing are unknown for now for an instance let’s assume that the edits work as intended.
This fact doesn’t change anything about the ethical impacts of gene therapy in general.
One of the most important effects of germline editing is that if it’s successfully implemented clinically, it might increase inequality among societies.
And no doubt human germline editing is going to be expensive, so it clearly suggests that rich peeps can afford it whereas poor or middle-class peeps can not but the usage of germline editing is completely hypothetical at this point.
Improving CRISPR Gene Editing Technology
CRISPR would surely be the gold standard in gene editing, but its ‘offset targeting’ is a setback. So there are certain methods for precisely implementing CRISPR without causing harm to the rest of the genome.
Choosing the sgRNA appropriately is one of the fundamentals for precision in CRISPR gene editing but how could you possibly do that knowing that DNA is similar at different sites within the genome?
That’s where machine learning enters the spotlight.
Machine Learning will Improve Precision in CRISPR Gene Editing
Computational techniques are typically employed to identify the most suitable sgRNA binding site in the target genome’s region of interest.
These technologies typically produce a huge list of offsite targets for each expected site in order to cover all potential offsite targets.
However, not all expected locations are cut in half in ‘in-vivo experiments’.
This technique frequently results in incorrect prediction of off-site targets and can even result in the omission of the most suited sgRNA.
Researchers claimed to have developed a machine learning-based computational model in a recent paper published in ‘Science Direct.’
In experimental trials, this model identifies potential off-site targets with a 91.49% accuracy. [9]
This model is based on the XGboost algorithm, which is an open-source implementation of the gradient-boosted tree technique.
The gradient-boosted tree technique predicts a target variable by merging estimates from weaker and simpler models.
Altering Cas9 Delivery
When using CRISPR, a single Cas9-sgRNA combination is employed to make changes to a specific spot of DNA. The severity of off-target edits can thus be lowered by utilizing a lower dose of CRISPR-Cas9 reagents.
Instead of plasmids, a group of researchers employed RNPs (Ribonucleotide Protein) to encode Cas9 reagents directly into cells by electroporation. [10]
Another group of scientists developed a Cas9 variant that can only cleave the DNA only in the presence of a certain molecule [4-hydroxytamoxifen]. [11]
These methods can limit off-target cleavage activity, however, they can also result in a decrease in on target frequency of DNA splicing.
Modification of Cas9 Enzyme
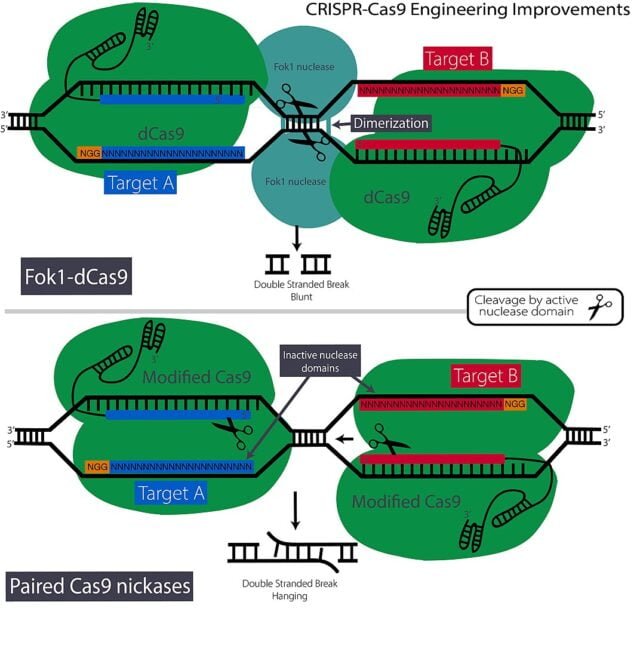
Another approach for precise CRISPR is by using “nickase” variants of Cas9.
Nickase variations are created by modifying one of the two Cas9 nuclease domains; nickase cuts DNA in a single-stranded form rather than dual-stranded cuts. [12]
When used with two adjacent sgRNAs it can lower the probability of off-site targeting of the DNA.
Unfortunately, this method has a serious backside as well because using nickase also reduces ‘on-site mutagenesis,’ and the necessity to discover two appropriate sgRNAs based on the intended site is a time-consuming task.
It also restricts the number of potential regions on DNA where you can use CRISPR on.
Future Applications of CRISPR Gene Editing
CRISPR gene editing has changed dramatically since its introduction as a rising star in molecular biology and gene editing, and it continues to change.
CRISPR technology has unprecedented power, altering the genome in a matter of months or days and, quite honestly, controlling the entire human species (not recommending you should, just a metaphor).
Only when we solve the problem of CRISPR’s “off-target activities.”
While working with CRISPR on people, the off-target consequences of CRISPR represent a significant risk to patient’s health and disease-related safety.
And when there is a risk that the mutation is potentially hazardous or life-threatening to the patient, things get a little twisted up.
Even in the context of research, off-target activity is very undesirable because it wastes time, money, and effort in laboratories, there are currently no rock-solid ways for mitigating the possibility of CRISPR offset activity.
However, work continues, and a mix of those strategies can be used to best utilize CRISPR and optimize the possibility for exciting discoveries using CRISPR-Cas9.
CRISPR Gene Editing’s Bright Future in Medicine
CRISPR is used for the treatment of various congenital diseases, rare genetic disorders, infectious diseases, and cancers too.
Specifically in cancer research, there are a lot of studies going on lung and breast cancer. It can be used to treat cancer by knocking out PD1 genes in lymphocytes, allowing them to identify and attack cancerous cells.
In the treatment of blood disorders, CRISPR can be used to modify bone marrow stem cells, turning on the BCL11A gene to produce high levels of fetal hemoglobin in red blood cells.
For Duchenne’s muscular dystrophy, CRISPR-Cas9 is used to conduct a selective cut at 12 hotspot locations, potentially covering the majority of the 3000 mutations causing DMD.
CRISPR was designed to snip out the defective CEP290 gene from the photosensitive rod cells in the retina of the eye, to treat congenital blindness.
Genetically Humanizing Animals – Xenotransplantation
CRISPR gene editing is also being used to genetically humanize animals for xenotransplantation, to reduce the risk of infection and organ rejection in the recipient.
Decrypting the human genome has always been a headache to scientists (at this point me too..) after 19 years of the completion of an extremely ambitious project to sequence the human genome.
We’re still clueless, so what to do to solve the endless quest of this genomic mystery?
We create models (yay!), and scientists create human models to study various diseases and of course our friendly neighbor our genomes.
We can create any human-based animal model more or less for our own vicious purposes (just kidding, there are good ones too) by using CRISPR (duh.)
One of the reasons we need to “humanize” animals is that organ donors are becoming more scarce.
The demand for life-saving essential organs significantly outnumbers the supply, and most patients die while waiting for the organs they require.
Xenotransplantation is a surgical operation that involves the transplantation and implantation of a non-human animal recipient.
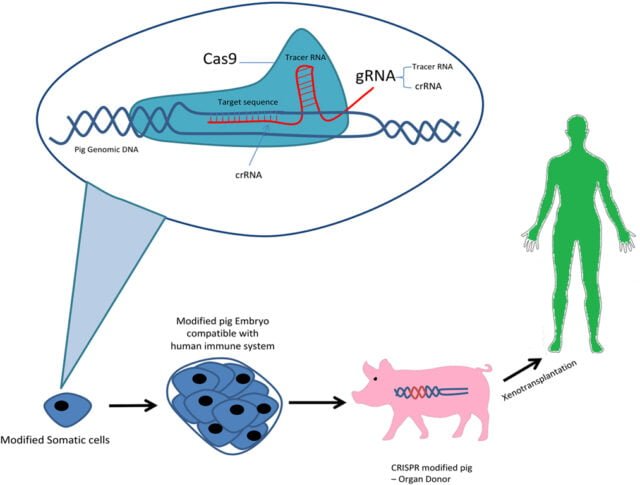
Although the potential benefits are clear, xenotransplantation raises significant worries about possible infection in the recipient.
With a possibility that the infectious agent may or may not be recognized, and the non-recognized agent can’t be recognized with current techniques, and a possibility that infection might transmit to the general human population.
And other concerns lead to possible organ rejection, which is also adamant with normal transplants but when you’re working with an animal recipient concern grows a little.
Wrapping up CRISPR Gene Editing
CRISPR is indeed a useful tool in genetic research that acts as molecular scissors, it can cleave DNA from any desired site.
While some of these treatments are still in the early stages, the potential of CRISPR gene editing has been shown to be a powerful tool in medicine with far-reaching implications.
We’ve explored numerous ways in which humans have revolutionized the biological world.
CRISPR gene editing has the potential to be used for treating genetic diseases, curing blindness, and even humanizing animals.
However, ethical considerations need to be taken into account when working with the CRISPR Gene Editing technology, there are flaws within the technology that need to be resolved before applying this technology to human beings and editing the human genome.
And one can say with complete certainty that CRISPR holds great significance in the future of medicine. Treatment of diseases like cancer and genetic disorders won’t be a problem anymore in the future.
And for some of you who might be wondering, no you can’t grow blades like Wolverine by modifying your genome using CRISPR :p
References
- ‘What are genome editing and CRISPR-Cas9?: MedlinePlus Genetics’, Medline Plus, n.d, “CRISPR-Cas9 was adapted from a naturally occurring genome editing system that bacteria use as an immune defense.”[↩]
- Amber Dance, ‘Core Concept: CRISPR gene editing’, PNAS, n.d, “The Cas9 enzyme cuts DNA at a specific sequence, determined by an accompanying bit of RNA called a guide RNA. Then, the cell’s own DNA repair machinery typically takes over in one of two different modes.”[↩]
- ‘Questions and Answers about CRISPR | Broad Institute’, Broad Institute, n.d, “CRISPR-Cas9 system itself is capable of cutting DNA strands, CRISPRs do not need to be paired with separate cleaving enzymes.”[↩]
- Su Bin Moon et al. ‘Highly efficient genome editing by CRISPR-Cpf1 using CRISPR RNA with a uridylate-rich 3′-overhang | Nature Communications’, Nature, 7 September 2018, “CRISPR-Cpf1 is an RNA-guided, class II CRISPR/Cas system that is analogous to CRISPR-Cas9, but shows unique features distinct from those of the CRISPR-Cas9 system.”[↩]
- Puping Liang et al., ‘CRISPR/Cas9-mediated gene editing in human tripronuclear zygotes | SpringerLink’, Springer Link, 18 April 2015, “Genome editing tools such as the clustered regularly interspaced short palindromic repeat (CRISPR)-associated system (Cas) have been widely used to modify genes in model systems including animal zygotes and human cells.”[↩]
- ‘The Woolly Mammoth Revival’, Revive&restore, “The genes of woolly mammoth traits can be edited into the Asian elephant genome.”[↩]
- Dr. Damiano Rondelli, ‘CRISPR technology to cure sickle cell disease‘, Science Direct, “A new article reports two patients to appear to have been cured of beta-thalassemia and sickle cell disease”[↩]
- Francoise Baylis, Marcy Darnovsky, Katie Hasson and Timothy M. Krahn, ‘Human Germline and Heritable Genome Editing: The Global Policy Landscape | The CRISPR Journal’, 20 October 2020, “When genetic changes are made to in vitro early-stage embryos, gametes (eggs and sperm), (…) the descendants would inherit a genetically modified genome.”[↩]
- Jaspreet Kaur Dhanjal et al., ‘Evaluation of off-targets predicted by sgRNA design tools’, Science Direct, 9 July 2020, “Out of all the supervised learning algorithms used to train the classifiers, gradient boosted regression trees (xgboost) resulted in predictions with the highest accuracy of 91.49%.” https://www.sciencedirect.com/science/article/pii/S0888754319307086[↩]
- Sojung kim et al., ‘Highly efficient RNA-guided genome editing in human cells via delivery of purified Cas9 ribonucleoproteins’, Cold Spring Harbour Laboratory Press, 24 June 2014,p.[5], “To sidestep these limitations, we delivered RGEN RNPs, rather than plasmids, directly into cells via electroporation.” https://genome.cshlp.org/content/24/6/1012.full.pdf[↩]
- Kevin M. Davis et al., ‘Small molecule-triggered Cas9 protein with improved genome-editing specificity’, PubMed, 11 May 2015, “We developed Cas9 nucleases that are activated by the presence of a cell-permeable small molecule by inserting an evolved 4-hydroxytamoxifen.”, https://pubmed.ncbi.nlm.nih.gov/25848930/[↩]
- Mary Gearing, ‘Cas9 Nickase Design and Homology Directed Repair’, Addgene, 15 March 2018, “Nickases create a single-strand rather than a double-strand break, and when used with two adjacent gRNAs, can lower the probability of off-target editing.” https://blog.addgene.org/crispr-101-cas9-nickase-design-and-homology-directed-repair[↩]